“Net zero nets benefits” is a catchy slogan but where does the term net zero come from and what does it mean?
The Paris Agreement limiting global warming was negotiated by 196 parties at the 2015 United Nations Climate Change Conference near Paris, France. As of February 2023, 195 members of the UNFCCC (United Nations Framework Convention on Climate Change) are parties to the agreement. Of the three UNFCCC member states which have not ratified the agreement, the only major emitter is Iran. The United States withdrew from the agreement in 2020 under President Trump but rejoined in 2021 under President Biden.
The Paris Agreement’s long-term temperature goal is to keep the rise in mean global temperature to well below 2 °C (3.6 °F) above pre-industrial levels, and preferably limit the increase to 1.5 °C (2.7 °F), recognizing that this would substantially reduce the effects of climate change. Emissions should be reduced as soon as possible and reach net zero by the middle of the 21st century. To stay below 1.5 °C of global warming, emissions need to be cut by roughly 50% by 2030. This is an aggregate of each country’s nationally determined contributions.
To meet this goal, the United States established an ambitious agenda to reduce net GHG (greenhouse gas) emissions by 50–52% from 2005 levels by 2030 and to reach net-zero emissions economy-wide by no later than 2050; including a goal to reach 100% carbon-free electricity by 2035.
Statistically, the nation is slowly achieving the early benchmarks necessary to reach the 2050 goal. A major segment of the economy, construction and construction materials, and its corollary existing buildings, are a driving force in meeting the goal.
Netting Net Zero
Net zero refers to a state in which the greenhouse gases going into the atmosphere are balanced by removal out of the atmosphere according to Oxford Net Zero, an interdisciplinary research initiative based on the University of Oxford’s 15 years of research on climate neutrality. The term is important because—for CO2 at least—this is the state at which global warming stops. The Paris Agreement requires states to “achieve a balance between anthropogenic emissions by sources and removals by sinks of greenhouse gases in the second half of this century.”
So, to “go net zero” is to reduce greenhouse gas emissions and/or to ensure that any ongoing emissions are balanced by removals. The “net” in net zero is important because it will be very difficult to reduce all emissions to zero in the time needed. Besides deep and widespread cuts in emissions, we will likely need to scale up removal of carbon and CO2.
For net zero to be effective, it must be permanent, meaning that removed greenhouse gas does not return into the atmosphere over time, for example through the destruction of forests or improper carbon storage. In fact, permanent or hard net zero refers to a balance between all greenhouse gas sinks and sources that is sustained over matching time scales.
Research is, of course, ongoing to find new ways to decarbonize construction materials and processes. Steel, cement, and chemical manufacturing are especially difficult industries to decarbonize, as carbon and fossil fuels are inherent ingredients in their production. Technologies that can capture carbon emissions and convert them into forms that feed back into the production process could help to reduce the overall emissions from these “hard-to-abate” sectors.
But thus far, experimental technologies that capture and convert carbon dioxide do so as two separate processes, that themselves require a huge amount of energy to run. A team at MIT is looking to combine the two processes into one integrated and far more energy-efficient system that could potentially run on renewable energy to both capture and convert carbon dioxide from concentrated, industrial sources.
The researchers published the hidden functioning of how carbon dioxide can be both captured and converted through a single electrochemical process. The process involves using an electrode to attract carbon dioxide released from a sorbent, and to convert it into a reduced, reusable form.
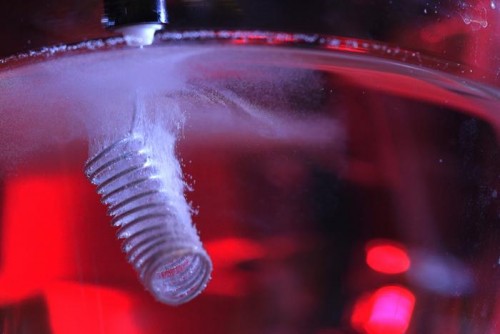
Others have reported similar demonstrations, but the mechanisms driving the electrochemical reaction have remained unclear. The MIT team carried out extensive experiments to determine that driver, and found that, in the end, it came down to the partial pressure of carbon dioxide. In other words, the purer carbon dioxide that makes contact with the electrode, the more efficiently the electrode can capture and convert the molecule.
Knowledge of this main driver, or “active species,” can help scientists tune and optimize similar electrochemical systems to efficiently capture and convert carbon dioxide in an integrated process.
What’s in a Word?
Different terms (carbon neutral, net zero, climate neutral) point to different ways in which emissions sources and sinks are accounted for in context. They help to indicate what is and is not included in the calculation or a target. Net zero is the internationally agreed upon goal for mitigating global warming in the second half of the century. The IPCC concluded the need for net zero CO2 by 2050 to remain consistent with 1.5C.
The U.S. has recommitted to reaching net-zero emissions by 2050. To accomplish this goal, large cuts in emissions are necessary, especially in high-emission sectors like the building industry. Recently, a team of researchers use a computational model to analyze several scenarios of future building energy use in the U.S. They found by tackling emissions on multiple fronts and placing focus on demand-side measures that affect how power is drawn from the grid, such as technologies like electric heat pumps and smart thermostats, the United States can achieve its climate goals, decrease building emissions by 91% from their 2005 peak, and save more than $100 billion each year on energy costs.
The team of energy technology experts based at LBNL (Lawrence Berkeley National Laboratory) advise, “Meeting the U.S. 2050 net-zero emissions target requires a rapid and cost-effective low-carbon transition across the entire energy system. Commercial and residential buildings are a primary source of emissions and are key to this transition.”
In the United States, buildings—including both public buildings, like offices, and private buildings, like homes—contribute 35% of the country’s total greenhouse gas emissions. In 2005, the U.S. contributed 2,327 megatons of carbon dioxide in the buildings sector, setting a record for all-time high emissions. Since then, emissions have declined by 25%, and are projected to keep declining by up to another 41% by 2050. But there is a continuing need to keep cutting emissions to reach the established climate goals.
Retrofitting buildings using a digital-first approach is perhaps the best pathway to decarbonization, according to research from Schneider Electric, a firm specializing in digital transformation of energy management and automation. The company’s findings show deploying digital building and power management solutions in existing office buildings could reduce operational carbon emissions by up to 42% with a payback period of less than three years.
If fossil fuel-powered heating technologies are replaced with electric-powered alternatives, and a microgrid with local renewable energy sources is installed, all-electric, all-digital buildings will see an additional 28% reduction in operational carbon emissions resulting in a total reduction of up to 70%.
The research, carried out with the global design firm WSP, is based on modeling the energy performance and carbon emissions of a large office building built in the early 2000s across various U.S. climate zones. This digital approach to building renovations is, however, applicable to all building types and climates, and is, therefore, one of the most effective decarbonization strategy, yielding fast results with lower “upfront carbon.”
Renovating through the deployment of digital technologies is not only less disruptive to daily operations, but also more effective from a lifecycle carbon perspective. Failing to rapidly decarbonize buildings could also result in stranded assets that lose value and are unattractive to both investors and tenants.
In addition, the LBNL team defined three main ways to cut building-related emissions: Focus on making buildings use energy more efficiently, make the power grid more reliable by increasing the flexibility of how energy is managed by the grid, and use low-carbon energy sources. The report claims, “Achieving deeper levels of emissions reductions will require a comprehensive mix of solutions addressing both the generation and end uses of energy—a true ‘all-of-the-above’ menu of solutions to decarbonize the built environment.”
The team modeled “low,” “moderate,” and “aggressive” scenarios of this “all-of-the-above menu” to determine the degree to which we can cut emissions. They found that it’s possible to reduce building emissions by 91% compared to 2005 levels by 2050, and that demand-side measures that increase the flexibility of the power grid, like heat pumps and smart thermostats, could contribute up to 45% of these emissions cuts.
However, achieving this level of emissions cuts would require the “aggressive” level of intervention from the researchers’ model, and the authors emphasize that an “unprecedented scale and speed of building technology development and deployment” would be necessary, as well as a largescale commitment to changing how we consume energy.
Realizing this level of change in the building sector will require a rapid and sustained increase in investment alongside policy and regulatory support and supply-side technologies to fulfill ambitious targets for climate change mitigation in the United States.
Picking on Buildings
Although building emissions today are 25% below their 2005 peak, far deeper reductions are needed to reach the 2050 net-zero emissions goal. While there are plausible decarbonization pathways that consider both buildings and their interactions with the power grid, they remain poorly understood. Detailed modeling of building energy use and the grid to quantify building decarbonization potential and associated grid impacts finds up to a 91% reduction in building CO2 emissions from 2005 levels by 2050 using building efficiency, demand flexibility, and electrification measures alongside rapid grid decarbonization.
Building efficiency and flexibility could generate up to $107 billion in annual power system cost savings by 2050, offsetting more than a third of the incremental cost of full grid decarbonization. This includes a goal to reach 100% carbon-free electricity by 2035. But achieving these goals will require unprecedented acceleration in the adoption of mitigation solutions across every sector of the economy.
Previous research on energy system decarbonization pathways has tended to focus on supply-side solutions for low-carbon energy generation and CO2 removal technologies rather than demand-side approaches, including those in buildings and other end-use contexts. Increasingly, however, studies suggest demand-side approaches are essential for climate change mitigation.
Building energy consumption is a substantial driver of the CO2 emissions from energy end-use sectors, accounting for 35% of the U.S. total. Buildings also account for 74% of annual U.S. electricity sales and 42% of end-use natural gas consumption. Total building energy-related CO2 emissions peaked in 2005 at 2,327 megatons CO2, the benchmark year for U.S. climate goals, and have declined by 25% in the 17 years since then.
Building decarbonization solutions improve the efficiency of energy end uses, flexibly manage building loads and other distributed energy resources to improve reliability of the power grid, and/or convert building services to low-carbon sources of electricity. Of the three approaches, building energy efficiency is the most extensively studied and widely considered as a beneficial, low-cost option for mitigating climate change, although its role is shifting alongside aggressive decarbonization of the energy supply.
Demand flexibility is a complementary solution that leverages demand-side assets such as smart thermostats, connected appliances, and behind-the-meter storage and generation to reduce peak building demand and shift demand to times of high renewable energy generation, flattening the overall shape of building demand on the grid. Flexibility will play an increasingly important role as variable renewable energy accounts for a larger share of power generation and distribution networks are challenged by growing demand for clean electricity.
Finally, building end-use EL (electrification) has emerged as a key pillar of economy-wide decarbonization, particularly as the cost and performance of EL technologies have improved while ambitious targets for power sector decarbonization have been announced. Recent research on pathways to economy-wide decarbonization in the U.S. represents building sector solutions as part of an accelerated transition and reveals a number of common themes.
First, final building energy demand is reduced significantly, up to 41% compared with business-as-usual in 2050. Second, rates of building space and water heating EL accelerate dramatically across studies: electric shares of new equipment sales in 2050 reach up to 90% for certain end-use segments, such as residential space heating. Third, regarding the power sector, studies assume a 70–100% reduction in fossil fuel use for electricity generation by 2050. Some studies assume an aggressive target of achieving carbon-free electricity by 2035.
In cases with aggressive grid decarbonization, remaining building emissions are due primarily to the assumption that full EL is not achieved across building end uses; these remaining emissions in the studies are offset by deployment of negative emissions sources to achieve net-zero emissions for the building sector.
Existing cross-sectoral decarbonization studies tend to represent building decarbonization solutions and adoption drivers with a coarse degree of detail and, while they calculate the total and net costs of deep decarbonization across sectors, the cross-sectoral studies do not undertake detailed cost modeling for the building sector or assess the implications of ambitious building technology deployment scenarios on the power sector. Recent studies focused on building sector GHG emissions demonstrate the potential for deep emissions reductions but have similar limitations to the cross-sectoral studies in their representation of technologies and assessment of power system cost impacts.
Based on current forecasting and state-of-the-art procedures, we are still a long way from net zero. Unfortunately, we are not a long way from 2035 and 2050. The construction, building management, and architecture industries have an obvious responsibility for maintaining the drive forward to net zero and pushing the boundaries that are evident now and in the future.
Want to tweet about this article? Use hashtags #construction #sustainability #infrastructure #netzero